Low sea levels = heavy carbon, light sulfur in seawater
- Low sea levels = more land space for plants = plants use lighter carbon = lighter carbon stuck on land = heavy carbon in the sea!
- Low sea levels = less habitat for sulfate-reducing bacteria = less light sulfur in pyrite = more light sulfur in the sea!
High sea levels = light carbon, heavy sulfur in seawater
- High sea levels = less space for plants = less light carbon uptake by plants = light carbon in the sea!
- High sea levels = expanded habitat for sulfate-reducing bacteria = more light sulfur in pyrite = less light sulfur in the sea!
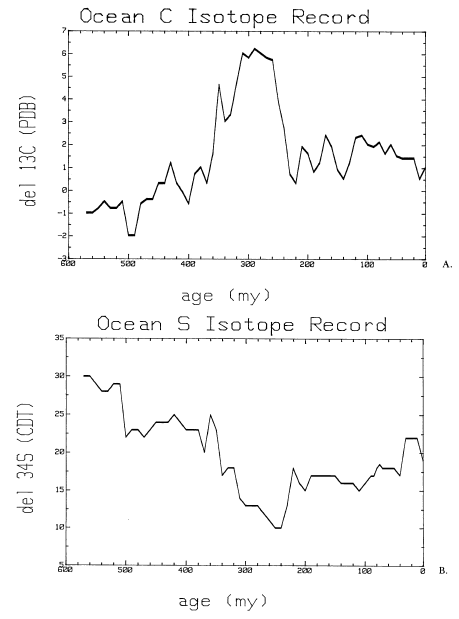
The stable isotopic composition of carbon (A) and sulfur (B) of the ocean through time. Credit: Kump, 1989.
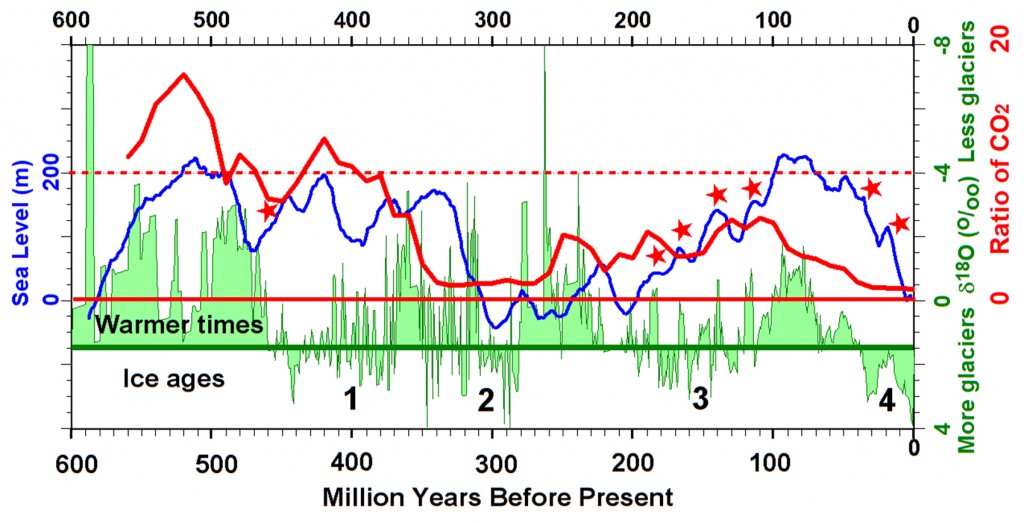
Sea level through time. Image credit: Peter L. Ward
Carbon isotopes and sea level
Plants preferentially uptake the lighter carbon isotope, carbon-12, as it forms weaker bonds and therefore requires less energy to remove it from inorganic reservoirs. Therefore, plants have a low δ13C. During times of low sea level, land space expands and accommodate additional terrestrial plants, resulting in greater storage of light carbon on land; thus, seawater becomes enriched (higher δ13C). During high sea level, the volume of terrestrial plants decreases as land is inundated, reducing terrestrial storage of light carbon and thereby allowing lighter carbon to reach seawater, which becomes depleted (lower δ13C). The δ13C of seawater can be calculated using the δ13C of ancient calcium carbonates, as calcifying organisms do not fractionate carbon when they precipitate shells/skeletons.
Sulfur isotopes and sea level
Reduced sulfur is largely stored in pyrite produced by the activities of sulfate-reducing bacteria. These bacteria live in fine-grained terrigenous sediments located along continental shelves, where upwelling and low-energy conditions allow the bacteria to thrive. The bacteria utilize organic material supplied by upwelling to reduce sulfate. One such possible reaction follows:
SO42- (aq) + CH3COOH (aq) + 2 H+ (aq) → HS- (aq) + 2 HCO3- (aq) + 3 H+ (aq)
At the same time, rivers provide the continental shelves with iron ions (largely Fe3+) stored in minerals such as biotite, hornblende, and garnet. Iron(III) and S2- react to form pyrite through a series of three steps, including two redox reactions (the first and last equations):
2 Fe3+ (aq) + HS- (aq) → 2Fe2+ (aq) + S0 (aq) + H+ (aq)
2Fe2+ (aq) + HS- (aq) → FeS + H+ (aq)
FeS (aq) + S0 (aq) → FeS2
Similar to plants, bacteria prefer to work with less energy-intensive lighter isotopes, and so they preferentially utilize sulfur-32 at the expense of sulfur-34. During times of higher tectonic activity, higher sea levels expand the breadth of continental shelves, thereby expanding the working space for sulfate-reducing bacteria. When sea level is high and these bacteria have more working space, the light sulfur stored in pyrite formed by bacterial activities is then unavailable to seawater, so seawater becomes enriched in heavier sulfur isotopes (higher δ34S). During low sea stands, shelves shrink and rivers plummet directly into the slope, respectively reducing and disturbing the bacteria’s habit, causing the sulfur isotopic composition of seawater to become depleted (lower δ34S) as the bacteria stop storing light sulfur in pyrite.
Redox reactions
Reduced | Oxidized | ||
Carbon | C0H2O (organics) + O2 | +← 4e- →- | HC4+O3- (reacts with calcite) + H+ |
Sulfur | S2- (in pyrite) + 2O2 | +← 8e- →- | S6+O42- (gypsum) |
Resources
Kump, L. R. (1989). Alternative modeling approaches to the geochemical cycles of carbon, sulfur, and strontium isotopes. Am. J. Sci, 289(4), 390-410. http://earth.geology.yale.edu/~ajs/1989
/04.1989.03Kump.pdf
Garrels, R. M., & Lerman, A. (1981). Phanerozoic cycles of sedimentary carbon and sulfur. Proceedings of the National Academy of Sciences, 78(8), 4652-4656. http://www.pnas.org/content/78/8/4652.full.pdf
Low sea levels = heavy carbon, light sulfur in seawater
- Low sea levels = more land space for plants = plants use lighter carbon = lighter carbon stuck on land = heavy carbon in the sea!
- Low sea levels = less habitat for sulfate-reducing bacteria = less light sulfur in pyrite = more light sulfur in the sea!
High sea levels = light carbon, heavy sulfur in seawater
- High sea levels = less space for plants = less light carbon uptake by plants = light carbon in the sea!
- High sea levels = expanded habitat for sulfate-reducing bacteria = more light sulfur in pyrite = less light sulfur in the sea!
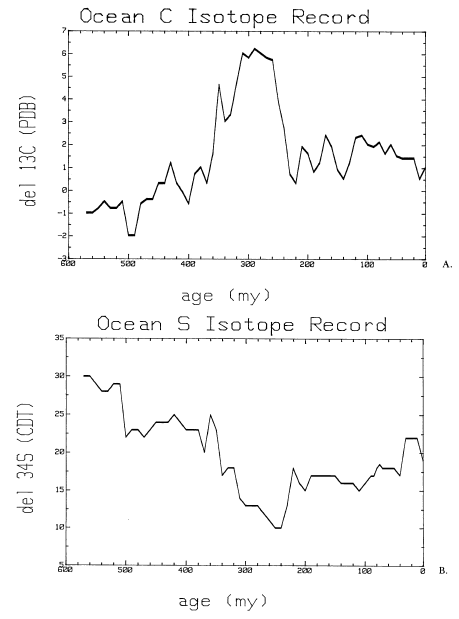
The stable isotopic composition of carbon (A) and sulfur (B) of the ocean through time. Credit: Kump, 1989.
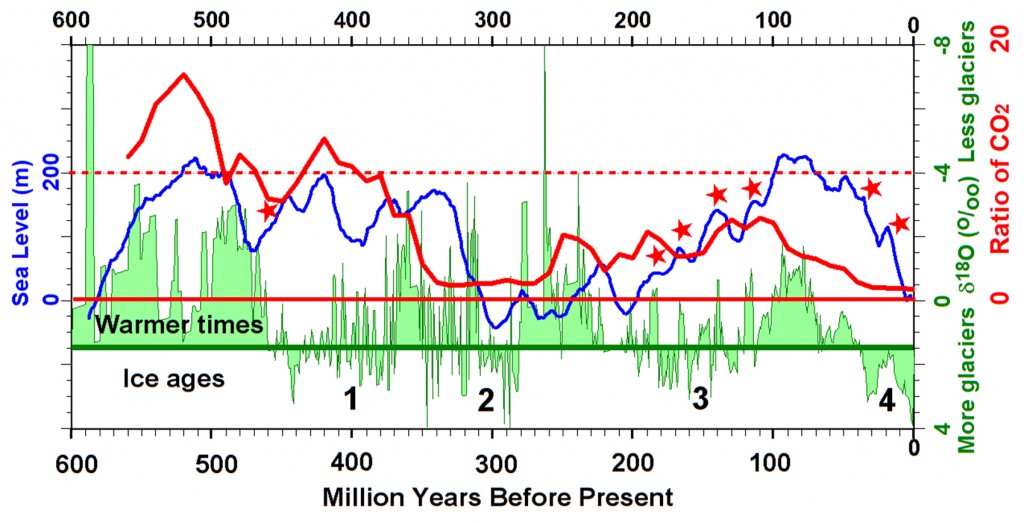
Sea level through time. Image credit: Peter L. Ward
Carbon isotopes and sea level
Plants preferentially uptake the lighter carbon isotope, carbon-12, as it forms weaker bonds and therefore requires less energy to remove it from inorganic reservoirs. Therefore, plants have a low δ13C. During times of low sea level, land space expands and accommodate additional terrestrial plants, resulting in greater storage of light carbon on land; thus, seawater becomes enriched (higher δ13C). During high sea level, the volume of terrestrial plants decreases as land is inundated, reducing terrestrial storage of light carbon and thereby allowing lighter carbon to reach seawater, which becomes depleted (lower δ13C). The δ13C of seawater can be calculated using the δ13C of ancient calcium carbonates, as calcifying organisms do not fractionate carbon when they precipitate shells/skeletons.
Sulfur isotopes and sea level
Reduced sulfur is largely stored in pyrite produced by the activities of sulfate-reducing bacteria. These bacteria live in fine-grained terrigenous sediments located along continental shelves, where upwelling and low-energy conditions allow the bacteria to thrive. The bacteria utilize organic material supplied by upwelling to reduce sulfate. One such possible reaction follows:
SO42- (aq) + CH3COOH (aq) + 2 H+ (aq) → HS- (aq) + 2 HCO3- (aq) + 3 H+ (aq)
At the same time, rivers provide the continental shelves with iron ions (largely Fe3+) stored in minerals such as biotite, hornblende, and garnet. Iron(III) and S2- react to form pyrite through a series of three steps, including two redox reactions (the first and last equations):
2 Fe3+ (aq) + HS- (aq) → 2Fe2+ (aq) + S0 (aq) + H+ (aq)
2Fe2+ (aq) + HS- (aq) → FeS + H+ (aq)
FeS (aq) + S0 (aq) → FeS2
Similar to plants, bacteria prefer to work with less energy-intensive lighter isotopes, and so they preferentially utilize sulfur-32 at the expense of sulfur-34. During times of higher tectonic activity, higher sea levels expand the breadth of continental shelves, thereby expanding the working space for sulfate-reducing bacteria. When sea level is high and these bacteria have more working space, the light sulfur stored in pyrite formed by bacterial activities is then unavailable to seawater, so seawater becomes enriched in heavier sulfur isotopes (higher δ34S). During low sea stands, shelves shrink and rivers plummet directly into the slope, respectively reducing and disturbing the bacteria’s habit, causing the sulfur isotopic composition of seawater to become depleted (lower δ34S) as the bacteria stop storing light sulfur in pyrite.
Redox reactions
Reduced | Oxidized | ||
Carbon | C0H2O (organics) + O2 | +← 4e- →- | HC4+O3- (reacts with calcite) + H+ |
Sulfur | S2- (in pyrite) + 2O2 | +← 8e- →- | S6+O42- (gypsum) |
Resources
Kump, L. R. (1989). Alternative modeling approaches to the geochemical cycles of carbon, sulfur, and strontium isotopes. Am. J. Sci, 289(4), 390-410. http://earth.geology.yale.edu/~ajs/1989
/04.1989.03Kump.pdf
Garrels, R. M., & Lerman, A. (1981). Phanerozoic cycles of sedimentary carbon and sulfur. Proceedings of the National Academy of Sciences, 78(8), 4652-4656. http://www.pnas.org/content/78/8/4652.full.pdf
Summarized from my handwritten notes taken during Dr. Terri Woods' GEOL 540 course at ECU in Fall 2014.